Neutron Activation Analysis
Neutron Activation Analysis (NAA) is one of the most sensitive methods used to measure the concentration of trace amounts of many elements in a variety of sample types. In NAA, a sample is bombarded with neutrons, resulting in the production of a radioactive isotope of the element of interest. Gamma rays emitted by the radioactive isotope are then analyzed. The energy associated with the radiation is characteristic of the radioactive isotope, and hence it is used for element identification i.e., qualitative analysis. The number of gamma rays emitted is correlated to the number of atoms present in the sample, i.e., quantitative analysis.
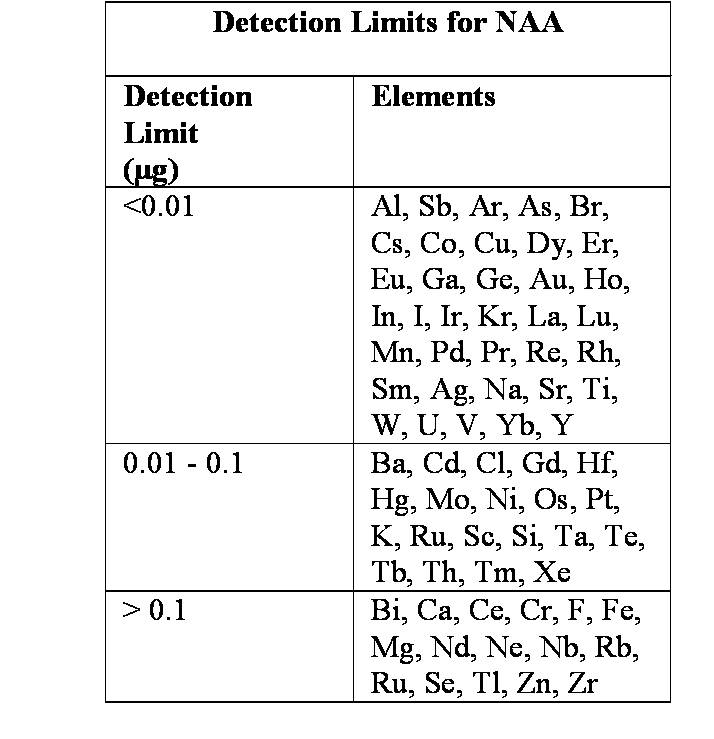
NAA is a microanalytical method; generally, only a few milligrams of sample are required. This is of value in examination of samples with limited amount of material such as archeological artifacts and criminal investigation evidence. NAA is a nondestructive technique, where analysis can be performed without destroying the sample. NAA is a multi-element procedure; multiple elements can be measured in the sample at the same time. Analysis can be performed on a sample as received. In most cases, neither chemical treatment nor addition of reagent is required to prepare the sample for analysis; thus, contamination from excess sample handling and reagent addition is eliminated.
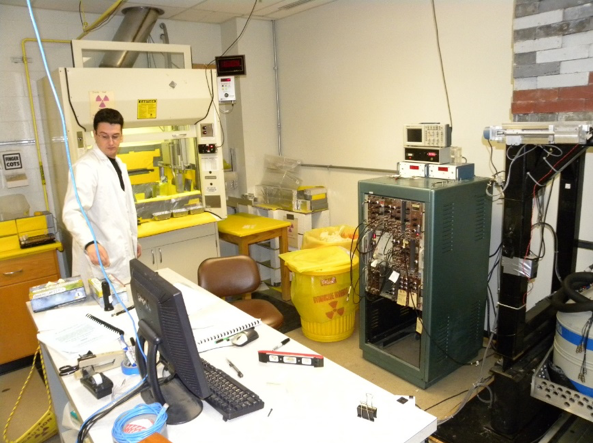
The irradiation time, decay time (time between irradiation and counting), and counting time are a function of the element to be measured and can be optimized to maximize the induced activity and minimize interference from other elements.
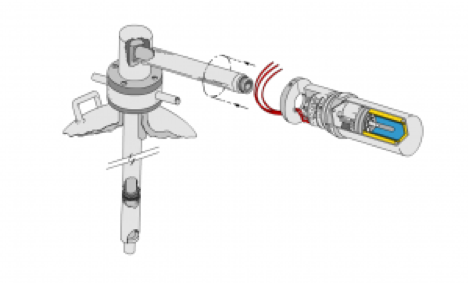
NAA has been in routine use to measure trace element concentrations in complex matrices such as human and animal tissue, coal, coal fly ash, petroleum products, river sediments, waste water, geological deposits, paper, air particulates, high purity metals, blood, urine, feces, sweat, tobacco, rocks, paint chips, animal feed, detergents and cereals.
Beam Port 1 (BP1): Cryogenic Irradiation Facility
The primary function of Beam-Port 1 (BP1) is to serve as a Cryogenic Irradiation Facility that can provide specific quantities of gas, at various levels of activation, for other experiments in a quick and efficient manner. This is achieved by sending a desired gas into a custom designed irradiation-condenser chamber inside BP1 while liquid helium is pumped down an adjacent line to cool the chamber from the outside. Having prior knowledge of melting and freezing temperatures of the gas and various rates of cooling and heating applied to the chamber, the gas can be condensed inside the chamber and await irradiation.
Beam Port 2 (BP2): Neutron Depth Profiling
Some elements produce charged particles with characteristic energy in neutron interactions.
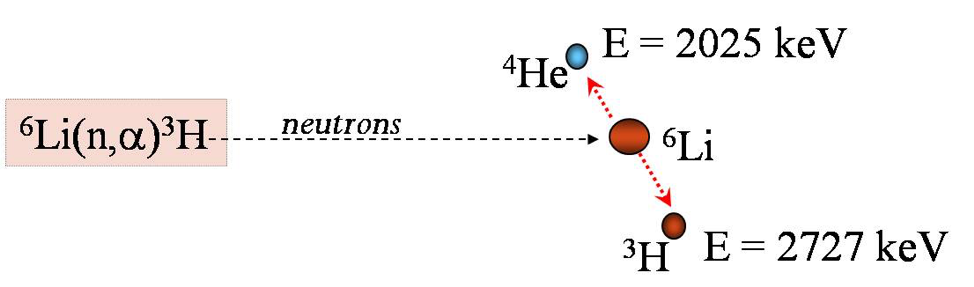
When these elements are distributed near a surface, the particle energy spectrum is modulated by the distance the particle traveled through the surface. NDP uses this information to determine the distribution of the elements as a function of distance to the surface.
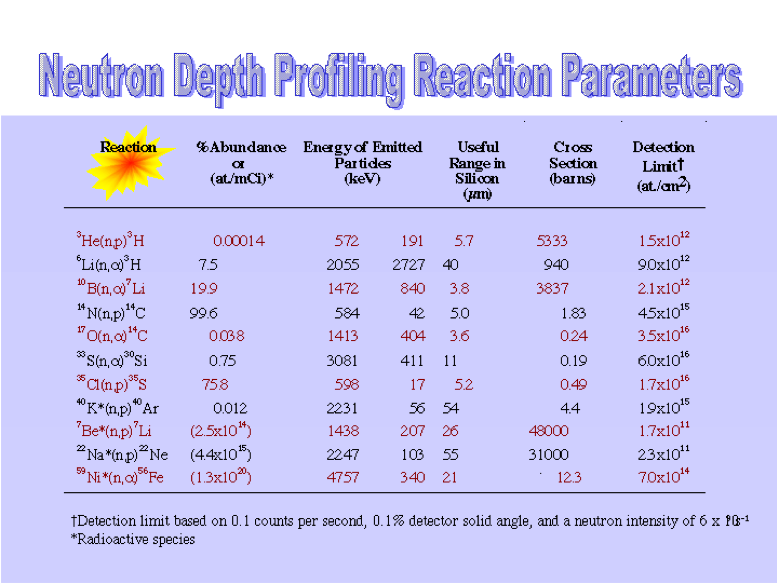
An illustration of equipment setup for NDP, with the beam exiting the reactor from the left and illuminating the sample in a vacuum chamber:
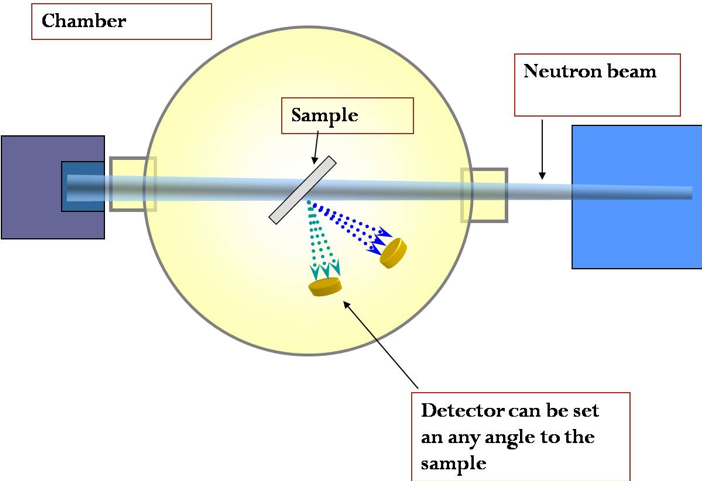
A spectrum generated by assessing a sample from the National Institute for Standards Technology:
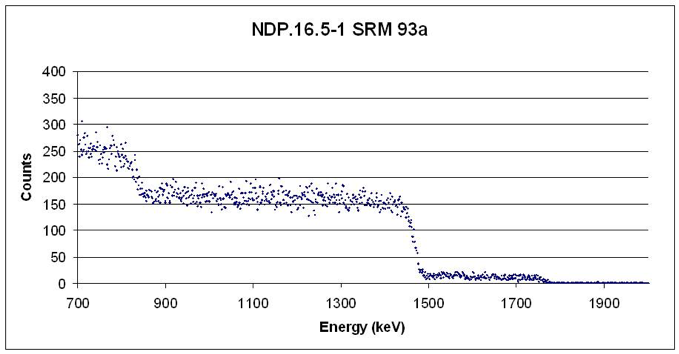
Beam Port 3 (BP3): Prompt Gamma Activation Analysis
Neutron capture prompt gamma activation analysis (PGAA) is a rapid, nondestructive, instrumental, nuclear technique that is used for trace and major component analysis of various elements.
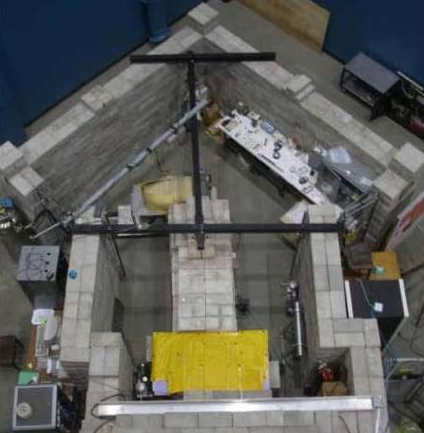
A dedicated facility for performing PGAA has been developed at the Nuclear Engineering Teaching Laboratory (NETL). In association with Neutron Activation Analysis (NAA), we have the capability of measuring the concentration of almost all elements.
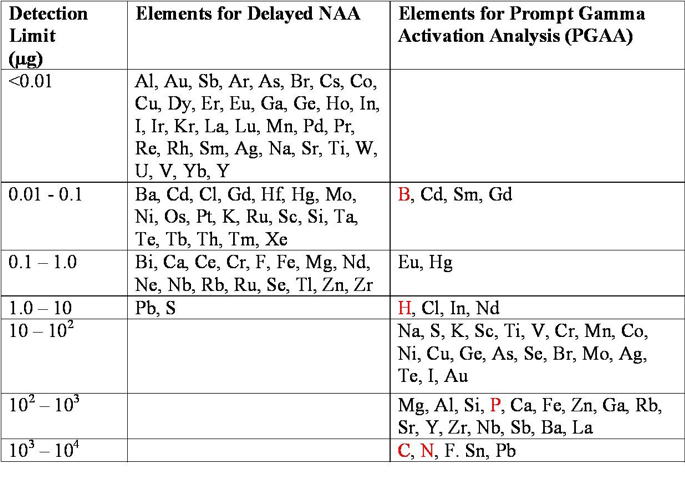
PGAA is based on detection of capture gamma rays emitted by a target material while it is being irradiated with neutrons. Nuclei formed in capture have excitation energies equal to the binding energy of the added neutron. The excitation energy is released by emission of gamma rays. The gamma-ray energies range from 100 keV to 10 MeV.
Nearly every neutron capture yields gamma rays that are potentially usable for analysis of the capturing element. However, the energy spectrum of capture gamma rays of most nuclides is complex. The capture gamma rays emitted by shielding material, etc., also complicate the analysis. Therefore, the relative merit of this technique for analysis of a particular element must be reviewed on a case-by-case basis.
PGAA is a nondestructive technique complementary to NAA and the analysis can be done in a relatively short time.. The advantages over NAA are as follows:
- PGAA is suitable for elements like B, H, and S that are difficult to measure via classic NAA.
- PGAA can often outperform NAA on biological samples due to the reduction of matrix interferences from Na, K, and Cl.
- Since PGAA irradiation is performed in beam lines external to the reactor core, this technique is more suitable for large samples in comparison to NAA.
The bulk of the applications of PGAA is the nondestructive multi-element analysis. Some examples of these applications are:
- Analysis of H in various H storage materials.
- Analysis of B and H in battery materials.
- Determination of the elements B, Cd, In, Hg, Mn, Fe, Co, Ni, S, and Cu in geological samples and environmental materials.
- Determination of the elements H, Cl, Na, K, B, Ca, P, and N in biological samples.
- Analysis of Fe, Cr, Ni, Mn, and B in stainless steel and iron ore samples.
Beam Port 4 (BP4): Fast Neutron Beam Irradiation Facility
The goal of this facility is to reduce the thermal and epithermal flux present in the neutron spectrum, while maintaining the fast flux to model the Watt-fission neutron spectrum. This was achieved using a variety of materials in a collimator-filter system design such as enriched boron and borated polyethylene. The result is a 10 cm diameter beam with an integrated flux of 6.63 x 105 n cm-2 s-1 at a reactor power of 950 kW. This economic facility has potential applications in studying fast-fission yields for nuclear forensics. This facility could provide improvements to fundamental nuclear data which in turn improves models for nuclear signature transport.
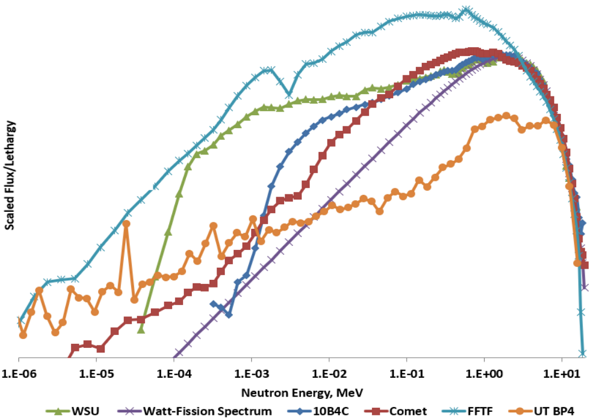
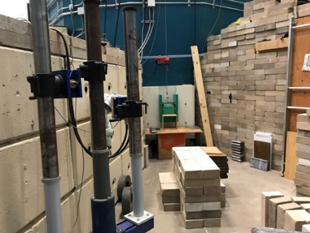
BP4 facility which consists of an external polyethylene and borated polyethylene shield to reduce dose within and in surrounding areas of the facility.
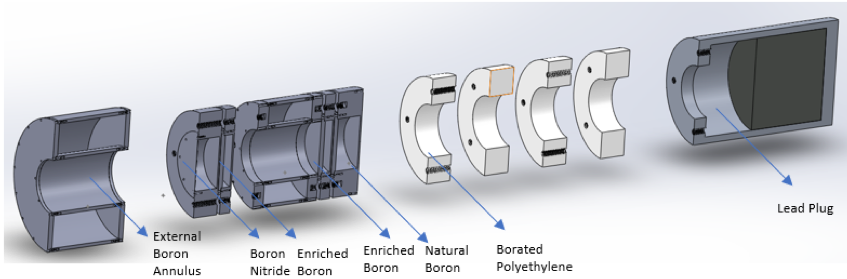
Current modular, collimator-filter system design following improvements based upon multiple sensitivity studies and design iterations.
Beam Port 5 (BP5): Neutron Radiography Beam
Neutron radiography uses the unique interaction probabilities of neutrons to create images of materials. This imaging technique is non-destructive; however, samples receive significant radiation exposure. X-rays are attenuated best by materials with large atoms and not well by small, light ones. This allows x-rays to pass through the water in a human body easily and attenuate in bones. Conversely, neutrons move through materials with large atoms easily and are attenuated well by materials with small, light atoms, such as water.
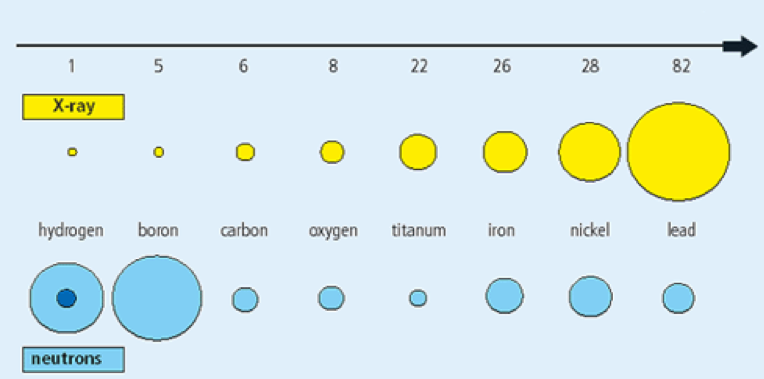
Much like x-rays, neutrons can be used to create images of the internal characteristics of an object. Neutron radiography is typically used to create images of non-hydrogenous materials since a neutron beam can pass through a significant amount of non-hydrogenous material without being completely attenuated. Materials made of heavier elements such as iron, silicon, or lead make good samples because changes in the thickness of these materials cause small but measurable changes in the neutron flux.
The neutron radiography system uses a high intensity, fast, collimated beam of neutrons. Samples are placed in front of a phosphor scintillation screen. Neutrons pass through the sample and strike the scintillation screen. Neutrons ionize the phosphorus in the screen, which cause it to produce flashes of light. The flashes of light are recorded by a camera and converted to numbers in a matrix.
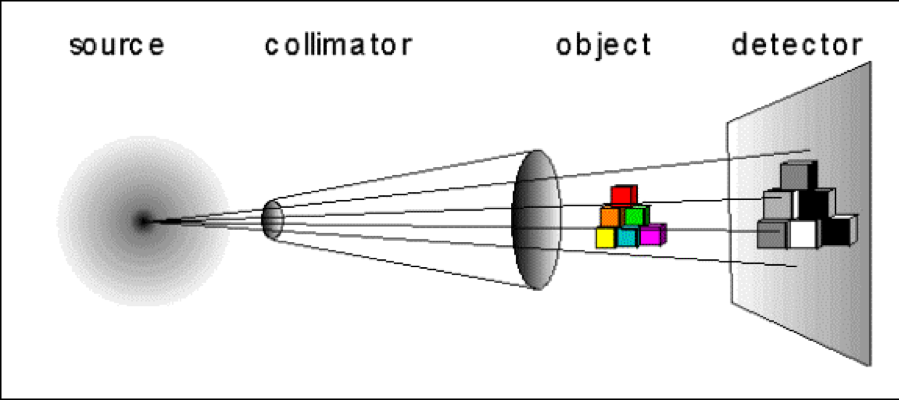
The neutron source is the TRIGA reactor with the collimator for these experiments in a beam port. The object in the figure above represents the sample that is to be imaged. The detector houses the scintillation screen, camera, and electronics to digitize the image and send it to the computer.
Longer exposure times increase the fidelity of the images. However, exposure times exceeding 10 milliseconds may lead to saturation, where entire sections of the image receive the maximum number of photons. In order to produce high fidelity images and circumvent saturation problems, several low quality 10 millisecond images are averaged together to create a single high-quality image. The image quality difference between images produced from 1, 10, and 100 ten millisecond snapshots is illustrated in the following figure.
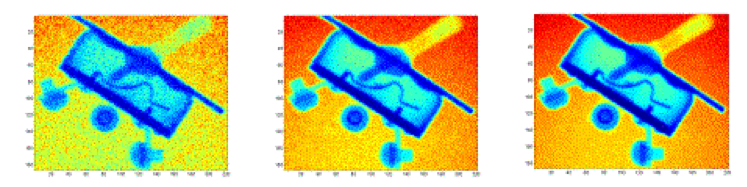
The images are typically produced by averaging several snapshots together. Radiography equipment is set up to generate digital files that each containing a matrix of numbers. The size of the numbers within the matrix is proportional to the quantity of photons emitted by the phosphorus screen at a given area. The digital files are processed to produce an image and can be enhanced to show specific details.